Wimshurst Generator
Landon Chamber’s group
The Wimshurst generator is a cleverly designed machine used to demonstrated static electricity in the late 19th and early 20th century. Two oppositely rotating disks spin against brushes, causing an excess charge on the metal sectors located on the disks. Collecting combs send the excess charges to two Leyden jars, one for positive charges and another for negative charges. One electrode is connected to each Leyden jar, and the ends of the electrodes are positioned closely together in front of the generator. Once the electric potential difference between the two electrodes is large enough, a visible spark lights up across the electrodes. Jake von Slatt, a Wimshurst generator hobbyist, captures the excitement of operating a Wimshurst generator with the following words. “The coolest thing about electrostatic machines is that you can feel them working. As you begin to crank a Wimshurst machine you will hear it start to crackle and hiss with energy, you will smell the sharp scent of ozone produced and you’ll feel the hair on your arm stand up as the Leyden jars charge.”
"Ion" Trap Demo
Michael Mehlman’s group
We have built a radio frequency quadrupole ion trap that can be used to confine charged corn starch particles. It consists of four rods along an axis that take oscillating voltages, with adjacent rods being out of phase http://www.triumf.ca/sites/default/files/images/RFQ-two_sections.png.
The trap has adjustable frequency up to 200Hz and adjustable voltage up to 12,000 volts! Charged corn starch dust likes to be trapped at around 60Hz and 10,000 volts. This is the same type of trap that is currently being used for nuclear physics research at the Cyclotron Institute.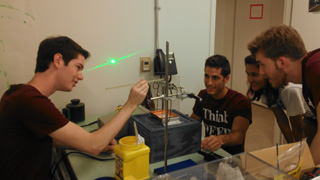
Archimedes Principle and the Power of the Buoyancy Force
John Mason’s group
Archimedes Principle states that the upward buoyant force that is exerted on a body immersed in a fluid is equal to the weight of the fluid that displaces. We are working on a demonstration that will show Archimedes Principle in different fluids while simultaneously eluding to the significance of the buoyant force. We will construct two cylinders filled: one filled with oil and one filled with water. Each cylinder will contain a bowling ball attached to a pulley. The displaced fluid should be different in each of the fluids. Furthermore, individuals will have the opportunity to lift the bowling balls out of each of the fluids. They will notice that the difficulty to lift the bowling bowl is much more difficult when they reach the air-fluid interface.
Aurora Simulator/Terrella
Leo Alcorn and Peter Chi’s group
A terrella is a small sphere that demonstrates how the charged particles from the solar wind interact with Earth’s magnetosphere. This demonstration uses a vacuum chamber with a trace amount of nitrogen that simulates the conditions in the upper atmosphere. A flat electrode inside the chamber acts as the solar wind, emitting charged particles. Moving lights are created by the transfer of energy between the charged particles and the low-pressure nitrogen. These lights simulate real aurora.
Balloon Paradox
Ellie Figueroa’s group
In this experiment, we allow a balloon filled with air to drop from the top of a transparent box while a balloon filled with helium floats up from the bottom. We then move the system horizontally and observe the motion of both balloons. While both balloons are expected to drift towards the rear of the box, we can see the helium balloon drift towards the front – the same direction as the box’s movement. This counterintuitive movement of the helium balloon is due to the compacted air molecules toward the rear of the box exerting a buoyancy force on the balloon, forcing it to move in the same direction as the applied force.
Bicycle Wheel Gyroscope
John Mason’s group
We will attempt to make a bicycle wheel gyroscope that can be turned using a mechanical crank. The bicycle wheel is suspended from one of its ends bye a rope. The wheel is then spun vigorously and allowed to hang by the end of the rope. The gyroscope levitates due to the torque created by the spinning wheel. This torque cancels the torque exerted on the wheel from gravity.
Bicycle Wheel Gyroscope
Timur Akhmedzhanov’s group
Bicycle wheel gyroscope is an amazing demo: just hang the wheel on the rope, rotate it fast by your hands and then turn it vertically. You’ll see that the wheel is literally defying gravity: instead of falling down, it starts to rotate slowly around the rope! It can be explained in terms of law of change of angular momentum: the wheel has a large angular momentum directed along its axis; gravity force creates horizontal torque that is perpendicular to the wheel axis and, thus, cannot turn the wheel down, but rather starts its slow rotation around the rope (precession of gyroscope!).
Black Box
Mariia Shutova’s group
This demonstration clearly shows how black body radiation works. The black box with a black hole in it looks like an ordinary box, but if you open it you will see that the box is painted white inside!
Blast from the Past: Ancient Greek Hydraulics
Sean Downes’s group and Tristan Leggett’s group
Many of the technological advances we enjoyed over the last century were first invented over two thousand years ago. Sadly, most of the technology was lost to history. It took humanity over a millennium to restore what was lost. Manuscripts that survived the destruction of the Library of Alexandria gave us insight into what the ancients were working on. Heron of Alexandria is credited with inventing most of what today we would call hydraulics.
Hydraulics is the use of fluids to do mechanical work. From construction equipment to gear shifting in performance automobiles, hydraulic technology surrounds us! Modern equipment uses synthetic chemicals and gasoline motors. In ancient times, they only had gravity, air and water.
In these demonstrations, we bring this ancient technology to life by applying modern design techniques to some of Heron’s devices. In the end, however, you can enjoy the simplicity of technology which is still only powered by gravity and runs on air and water.
Brazilian Nut Effect
Dawson Nodurft’s group
We demonstrate what is known as the Brazilian Nut Effect. When granular materials are subjected to vibration the largest particles end up on the top of the surface. This effect is dependent on volume, density, pressure and the shape of the container. Other phenomena that play a role in our demonstration include convection, percolation, and dilatancy.
Chladni Plate
Jacob Gayles’ group
Ernst Chladni, the father of modern acoustics, utilized Robert Hooke’s previous experiments of studying vibrating plates. When sand is sprinkled onto a bowing plate eloquent patterns emerge that are related to the physical dimensions and boundaries of the plate. Chladni plates, as they are known, help to visualize sound waves, which are undetectable by the human eye. Our demo is a modernized version of the Chladni plates.
Cloud Formation
Michael Cone’s group
Clouds form due to the condensation of water vapor in the atmosphere into small liquid water droplets. This happens due the adiabatic cooling effect that occurs when air containing large amounts of water vapor rises in the atmosphere. The pressure steadily drops with increasing altitude, and thus the air expands and cools. If the air is cooled below its dew point and becomes saturated, the water vapor can then condense to form a cloud. This demonstration shows this process by rapidly reducing the pressure inside a bottle with alcohol vapor inside, as the pressure decreases the alcohol rapidly condenses to form a cloud.
Cloud in a Bottle
Sasha Zhdanova’s group
By attaching a bicycle pump to a bottle filled with a small amount of water, closing it tightly, and pumping air, the molecules in the bottle are forced to squeeze together. The pressure builds up in the bottle, and when the cap is opened, the air rapidly expands. When air expands, it gets cooler, and the water vapor in the air condenses into tiny droplets to suddenly form a visible cloud. This is the same physics that causes clouds to form on Earth – when warm air rises, it cools and expands, and the water vapor that it carries becomes clouds. We use isopropyl alcohol here instead of water, because as a liquid with weaker bonds between its molecules, it evaporates more easily and makes thicker clouds. If the bottle is quickly re-pressurized, the cloud instantly disappears, because the building pressure warms up the air and causes it to reform as liquid at the bottom of the bottle.
Cognitive Illusion
Elizabeth Sooby’s group
This demo illustrates how the brain and eyes make unconscious inferences. Several frames of an animation are cut into thin, equally spaced lines and superimposed upon each other to make a single blurry image. Black bars are printed on a transparent overlay grating with the same spacing. When the grating is placed on top of the composite image, the black bars on the grating cover the spaces in the image and only one frame of the animation can be seen. When the grating is moved, the bars cycle through each frame of the animation, which the brain then interprets as one continuous movement.
Cornstarch
Jacob Gayles’s group Elizabeth Sooby’s group
The cornstarch demo is a favorite of the physics festival; where kids can physically feel the transition of a liquid into a solid. Mixing cornstarch with water results in a non-Newtonian fluid, defined by the properties changing based on forces applied. In this demo students get to play with the “oobleck” as it is called, see it dance on speakers and even run a pool of it. These non-Newtonian fluids are seen in everyday materials, such as ketchup, toothpaste, paint and blood. It also has applications in bulletproof vests, athletic shoes, and four wheel driving.
Cornstarch Speaker
Coupled Oscillators
A. Ryan Kutayiah’s group
This involves two masses on two separate strings hanging from a thin rod. As each mass oscillates as a simple pendulum, they become coupled by vibrations of the rod. This project is a playground for some interesting physics such as resonance and synchronization, that we encounter in a variety of both natural phenomena and man-made devices.
Curie Magnet
Jacob Gayles’s and Sriteja Upadhyayula’s groups
The idea of the Curie magnet demo is to show that all magnets have a specific temperature, above which they loose all their magnetism. This process can be reversed by lowering the temperature below this Curie point and causing magnetism to return to the system. This process can be seen when a magnet such as MnAs or Gadolinium is hung from a pendulum and at the low point in the pendulum there is a cooling source (dry ice). The dry ice causes the magnet to be attracted to a magnet off to the side. The magnet on the pendulum then heats up and looses its magnetism at temperatures higher than room temperature. The pendulum then returns to the low point and again is cooled.
Curie Temperature Demo
Sriteja Upadhyayula’s group
The Curie temperature (Tc) is the critical temperature beyond which a previously ferromagnetic material becomes paramagnetic. On the atomic level, below the Curie temperature the magnetic moments, contributed mainly by the electrons, are aligned in their respective domains and even a weak external field results in a net magnetization. As the temperature increases to Tc and above however, fluctuations due to the increase in thermal energy destroy that alignment. Tc for nickel is 631 K, while that for iron is 1043 K. This effect will be demonstrated by employing a nickel wire (made into a paperclip), and an iron one as well.
Curie Temperature Demo
Sriteja Upadhyayula’s group
The Curie temperature (Tc) is the critical temperature beyond which a previously ferromagnetic material becomes paramagnetic. On the atomic level, below the Curie temperature the magnetic moments, contributed mainly by the electrons, are aligned in their respective domains and even a weak external field results in a net magnetization. As the temperature increases to Tc and above however, fluctuations due to the increase in thermal energy destroy that alignment. Tc for nickel is ~631 K, while that for iron is ~1043 K. This effect will be demonstrated by employing a nickel wire (made into a paperclip), and an iron one as well.
Deceptive Reflection
Ellie Figueroa’s group
How can a head be apparently dismembered from its body? Through use of the laws of reflection we demonstrate the physical concepts in illusions such as dismemberment. We place a mirror set under a table to reflect an image at a certain angle away from the viewer. A volunteer then sits behind the mirror and puts his head up through a hole cut into the tabletop. Because of the mirror, this produces the illusion of decapitation.
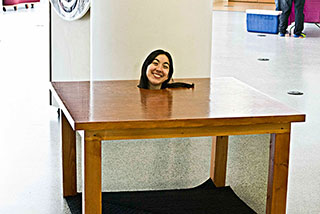
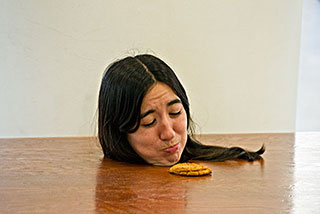
Diddley Bow – a Single String Guitar
A. Ryan Kutayiah’s group
Our diddley bow will be electric. For this project our group intends to build a speaker from scratch. We hope to use this speaker to play the sound generated by the diddley bow. The aim of the project is to demonstrate some basic physics concepts like standing waves, Faraday’s law of induction, transduction, etc. by using objects that people are familiar with.
Drawdio
Tyler Morrison’s group
Originally created by Jay Silver at MIT Media Labs. The Drawdio (Draw + Audio) is a fun way to connect sound with electronics. Based on the simple 555 timer the drawdio brings your drawings to life with sound. Building these with undergrads help them understand circuit design while creating something fun to share with anyone.
Electromagnetic Acceleration
Jonathan Perry’s group
For millennia humanity has employed the principles of physics to extend the range and improve the accuracy of their projectiles. Originally, as far back as 30,000 years, early hunters employed a simple lever arm action to throw a spear at nearly 100 mph (this device is known as the atlatl). As history progressed more complex physical principles were employed; the most commonly known of which are the bow and arrow, which employs spring potential energy, and the firearm or cannon, which uses pressure and impulse to move a projectile. The applications of these principles are still found in many places in today’s world in the military (weapons, missiles, etc), science (NASA), and even in the consumer world (paint ball!).
At the turning of the 19th into the 20th century, another method of launching projectiles was envisioned which exploits the use of electromagnetism. It was discovered that a magnetic field is generated when an electric current passes through a wire. This induces a force which causes the object to accelerate.
Thus the concept of a rail gun was born. A rail gun consists of two parallel conducting rails with a conducting projectile in between and in contact with both. The advantage of a rail gun over traditional, chemical projectiles is that it can launch projectiles at a much higher velocity (10’s of km/s). Drawbacks include material limitations and degradation of the rails after repeated launches.
Electromagnetic Can Crusher
Austin Baty, Elizabeth Sooby
We aim to construct a large capacitor bank capable of storing a large amount of energy, on the order of 10 kJ. By discharging the capacitors through a large coil of wire extremely quickly, we will be able to achieve an extremely large magnetic field strength at the center of the coil due to electromagnetic induction. If an aluminum soda can is placed inside the coil during the process, the can will be crushed inwards almost instantaneously by the strong magnetic forces it experiences. If we are able to get enough energy stored in the capacitors, it may be possible to get the can to rupture or be completely severed into two pieces.
Electrostatic Ping Pong
Dawson Nodurft
A neat way to observe the effects of electrostatics! See how a ping pong ball, covered in a magnetic surface, reacts when placed between two aluminum cans when an ion source is turned on!
Elliptical Pool Table
Joe Becker’s group
This is a novel pool table that demonstrates the unique properties of an ellipse. Rather than having pockets in the corners of a rectangle there is a a single pocket in a special location called the focus. An ellipse has two such points and when a ball is struck from the other focus it will go in the pocket no matter the direction it is struck.
Ferrofluid
Les Sheffield’s group
Ferrofluid is a colloidal suspension which means it is a material that displays the properties of two phases of matter. In the case of ferrofluid these properties are the magnetic properties of the solid metal, and the fluid properties of the liquid the nanoparticles are suspended in. We have several ferrofluid demonstrations including the “Magnetic Flower” which involves several large hidden neodymium rare earth magnets and a low friction transparent container, which holds the ferrofluid and allows viewers to witness it flowing freely along this surface and interacting with the hidden magnets. The “Climbing Liquid” involves a long test tube held above a pool of ferrofluid. This pool is held inside a large clear container, which seals to the test tube near its throat. Thus preventing any ferrofluid from escaping or leaking out of the container, which would be very messy! Viewers are invited to hold magnets along the side to drag the ferrofluid across the inside surface. A magnet on a string allows the liquid to -climb- up the side of the inner test tube all while making very interesting spiked patterns. This is a very interactive demonstration.
Fire Piston
Jacob Gayles’s group
The Fire Piston is a wonderful demo to show the thermodynamic property of changing the volume of a gas very quickly, which will change the temperature of the gas in the contained environment. This is the exact way a diesel engine works for ignition; with the exception that there is gasoline and air in the container. In our container there is air and a cotton ball. After increasing the temperature of the air to about 400 degrees Fahrenheit, the cotton ball will ignite. This demo shows about an 18:1 compression ratio of the air where the initial volume is about 9 ml.
Fire Tornado
Dawson Nodurft’s group
A combustible material, ethanol, is lit and placed on a rotating platform. The platform is enclosed with a screen, which creates a vortex of air inside. The rotating air reacts with the flame to create a tornado made of fire.
Hand Crank Cyclotron
Sriteja Upadhyayula’s group
This is a model of a cyclotron using Ping-Pong balls in a glass bowl being propelled around thanks to the repulsive forces of static electricity.
Hit The Target
Ellie Figueroa’s group
In this experiment we have setup a target which we expect to hit with balls launched by a gun. In some cases the target is allowed to fall as the ball is being launched, showing that the launched ball and the target fall at the same rate. In other cases the target is held stationary, forcing the gun to adjust it’s position to take distance and gravity into account.
Hoola Hoops
D. Heshani Jayatissa’ group
Hoola hooping is a perfect example of balancing different forces in nature by simply only using a person’s body. A person is able to keep a hula hoop spinning around the waist by applying an upward force and a turning force (torque). These two forces maintain the centripetal force of the hoop. While the weight of the hoop is trying to make it fall down due to gravity, the friction between the hoop and a person’s clothes help to maintain it at a uniform height. The exact force required depends on the size and weight of the hoop, as well as the size of the waist it is rotating around.
Human Sundial
Leo Alcorn and Peter Chi’s group
Human sundials allow us to tell time based on the length of our shadow. A person’s shadow will change length and position due to the changing position of the sun as the Earth rotates. The sundial is constructed by marking the ground for the times of the day where your shadow would fall when standing at a position for a given month, and is dependent on our latitude on Earth.
Imploding Watermelon
Ellie Figueroa’s group
By adding rubber bands around the watermelon, pressure is exerted on the watermelon causing it to implode.
Index Matching and Refraction
Michael Cone’s group
The index of refraction for a material is a parameter that describes the speed of light in the material. Specifically, it is the ration of the speed of light in the material to the speed of light in a vacuum. When light changes from one medium with a given index of refraction to another medium with a different index, the speed of the light changes, resulting in a change in direction for the light. This phenomenon is known as refraction, and is the basis for optical focusing elements like lenses. The amount of light that is reflected back off of a surface (e.g. the surface of a lake), also depends on the index of refraction. In particular, the larger the difference between the initial index of refraction, and that of the new medium (e.g. the index for air, and the index for water), the larger the amount of light that will be reflected back at the interface. This demonstration shows how changes this difference indices of refraction can alter how we see things, and how lenses work.
Kelvin’s Water Dropper
Andrew Traverso’s group
Kelvin’s Water Dropper is a very clever form of electrostatic generator. Water from a single source is divided into two separate streams which fall into two separate metal buckets. Each stream passes through a ring which is connected by a wire to the opposite side’s bucket, and there is no contact between the buckets, wires, or rings. When rods are connected to each of the buckets and the ends are held closely together, an electric spark jumps the gap. This is due to the fact that while water as a whole has no charge, there are individual positive and negative ions dispersed throughout it, although their charges usually balance each other out. When a charged object is placed near water, these ions form groups rather than intersperse throughout the water. Because of a slight difference in charge on each bucket to begin with, the ions in the water passing through the loop on one side separate so that they push electrons in the loop away from them, and therefore negatively charge the bucket to which it is connected. At the same time, the loop on the other side does the opposite and builds up positive charge. When the difference in positive and negative charges is great enough, electrons jump between the discharge rods to produce a spark and equalizes the charge.
Laser Game
Sasha Zhdanova’s group
This interactive and competitive game demonstrates the law of refraction, and how it can be used in laser optics. On the game board, there are two sets of laser rigs on top of a magnetic sheet, and the players position their mirrors on the sheet to point the laser into a sensor. The lasers will automatically shift position on a time interval, challenging the players to consider geometry and use their intuitive understanding of the physics of reflection in order to complete the laser’s path. For fun, the game is on a timer, and two players compete with one another to move their mirrors and deflect their laser onto the target the fastest.
Laser Light Show
Sasha Zhdanova’s group
If you play with a laser pointer, you might notice that sweeping it quickly across a flat surface makes it look a solid line, even though it is just a point. This is a trick of the eye, but it can be used to trick the eye very well.
By mounting a simple laser onto two small finely-tuned motors, it can be programmed to trace out a two-dimensional image onto the wall. Although the full image can clearly be seen, it is merely a single point moving quickly and precisely enough to fool the eye. The human brain can only process so many images per second, so if the laser picture is seen with a camera with a high enough frame rate, the picture is no longer clear. This demo can convert simple vector images drawn on the computer into laser light works of art.
Laser Sound Transmission
Andrew Traverso’s group
These days, lasers are used for data transmission through fiber optics. We demonstrate laser data transmission (music) through open air. This is accomplished by modulating the laser intensity. This is very similar to listening to how radio stations work. Radio is broadcast using either frequency modulation (FM) or amplitude modulation (AM), and in this demo we look at how AM works. AM works by varying the signal strength, and this varying specifies what sounds the speaker should produce. This demo is basically a small scale version of radio towers. Through the use of a laser we make it physically possible for people to see the transmission of the signal that we don’t normally see as well as how data transmission through fiber optics works as well.
Laser Waterfall
Alexx Perloff’s group
In this experiment we will fill a box with water and allow that water to drain out of a small hole in one of the sides. This will cause a spout of water to form. We will then point a laser at the hole in the box and the spout of water. If we hit the side of the spout at an angle greater than ~48 degrees, then the laser light will undergo total internal reflection. This means that the beam will be guided by the spout of water and will not continue along its original path.
Lens of Doom
Leo Alcorn and Peter Chi’s group
Astronomers are in the business of collecting as much light as possible. The larger the diameter of the telescope, the more light the lens or mirror can collect, and the deeper into space we can look. In this demo, we show the true power of a light collecting area using one of largest commercially accessible lenses: a big screen TV Fresnel lens. This demonstration shows how the large light-collecting area of a Fresnel lens can produce an intense magnification at the point where the light converges, the focal point. If we place objects at the focal point and aim the lens towards the Sun, the intense magnification can burn wood, boil water, or even melt copper. World-class modern telescopes, like the under-construction Giant Magellan Telescope partly funded by Texas A&M, are many times bigger than a Fresnel lens and use such power to observe the deepest objects in space.
Levitating Magnet
John Mason’s group
We will create a levitating magnet through the science of induce eddy currents. We will place a very strong magnet on top of a thick aluminum disk. The disk will be attached to a motor and rotated at high speeds. As the aluminum disk rotates, eddy currents are generated from the changing magnetic field of the magnet. Since these eddy currents oppose the magnetic field of the magnet, the magnet begins to float. Once the motor is turned off, the magnet returns back to the surface of the aluminum disk as no new eddy currents are created.
Lidar Demonstration
Andrew Traverso’s group
Light Detection and Ranging (LIDAR) is an optical remote sensing technology that is used to help measure the distance to a target by utilizing the pulses from a laser. Often, the LIDAR data is collected and analyzed in order to create 2D /3D maps of the immediate surroundings. This demonstration will feature a simple LIDAR system that will demonstrate what can be accomplished with a light sensor. A laser rangefinder mounted on a revolving base inside of a partially enclosed box will be used to collect distance data from moveable wooden blocks also inside the box. Viewers will have the chance to move around the blocks and see how the data output on the display changes in real-time as the LIDAR system scans the objects from one side to another.
Ligo
Dawson Nodurft
Ever wondered how gravity waves were detected? In this demo, we show a mini-version of the famed LIGO experiment. LIGO uses the amazing precision of light-interferometry to detect the smallest changes in the distance that light travels in the experiment.
Magnetic Levitation
Les Sheffield’s group
The magnetic levitation demo uses a high temperature superconductor called YBCO which exhibits superconductivity when cooled down to liquid nitrogen temperature (~77K). The Meissner effect expels all magnetic fields from the superconductor and induces the levitation. Flux pinning also occurs, essentially locking the superconductor in place above the magnets. That means that even if the system is inverted it will follow the track! This demonstration is truly amazing because the superconductor truly levitates above the track until it warms above the critical temperature and all superconducting properties disappear.
Magnetic Viewers
Tyler Morrison’s group
Magnetic Viewers are thin sheets of translucent material that are covered with micro sized capsules containing flakes of magnetic material suspended in oil. When brought into a magnetic field the small magnetic flakes will align parallel or perpendicular to the sheet. This causes the material to appear bright or dark respectively allowing one to “view” the magnetic structure of the magnetic field.
Magnetostriction
Tyler Morrison’s group
Some magnetic materials actually change their shape or size when magnetized. Dimensions of a rod of Terfenol-D are measured and compared before and after being magnetized with a strong magnetic field. When inside the magnetic field the Terfenol-D actually grows in size!
Make a Quake
Dawson Nodurft’s group
We demonstrate how seismic vibrations can be recorded by allowing you to make your own earthquake!
Making a Laser Beam Curve in a Sugar Solution
Michael Cone’s group
In this demonstration, laser light reflects off the surface of a flat mirror as it passes through a non-uniform sugar solution. After each bounce the beam curves in the sugar solution and comes back down to the mirrors surface. Why? Refraction explains the beams curved, skipping stone path. As light enters a different medium, it either speeds up or slows down depending on the medium’s composition. This change in velocity then causes the light to change direction, or to refract, as predicted by Snells law. Refraction due to a single change in medium is quite interesting, but if we instead have many changes in mediums the effect can be even more impressive. This demonstration involves a medium that constitutes a smooth composite of individual layers of differing concentrations of sugar. Because the sugar solution is allowed time to settle, the concentration of the solution increases gradually with increasing depth. Each level of concentration corresponds to a certain index of refraction, and as the laser light passes through each thin layer, the lasers trajectory is slightly adjusted. This steady gradient in the index of refraction causes the laser to trace out a continuous curve through the solution.
Methane Bubbles
Dawson Nodurft’s group
Using methane instead of normal air, we blow bubbles from an ordinary soap solution. The methane is less dense than air so bubbles made with it rise like a balloon filled with helium. Methane is a combustible gas though so when the bubble is exposed to a lighter the bubble bursts creating a large flame.
Metronome Synchronization
Jacob Gayles’s group
This synchronization of metronomes demonstration is a fascinating way to show a non-trivial behavior of a collection of simple mechanical systems when coupled through a small interaction. Synchronization is observed in everyday life such as the cells in the heart beating together or a group of people clapping together. Two metronomes are placed a top of a board with soda cans placed underneath in order for the board to roll. This causes the two pendulums to couple with two modes of oscillations, together/symmetric and opposite/anti-symmetric. It is found that the symmetric case is more stable because it is lower in energy at fixed amplitude and frequency of the oscillations. This demo is even more impressive if the number of metronomes is increased.
Microwave Grape Plasma
Sriteja Upadhyayula’s group
We create plasma with grapes by first cutting them in half, with the skin joining them still intact. This configuration acts as a good antenna for the microwaves, whose frequency is around 2.4GHz. This gives us a wavelength of around 12cm, translating into roughly 4x the grape’s length. This allows allows the power to focus in the middle of the two halves. The skin joining the two halves heat up, vaporize and burst into flame creating a plasma.
Newton’s Third Law Force Pair
John Mason’s group
Sit in for the greatest battle of Tug of War yet! Try to best your opponent by beating them in a battle of cart tug of war. Simply sit on the cart and try to pull your opponents cart across the boundary line. However, you will meet some unexpected foes along the way. Newton’s third law, which states that every action has an equal and opposite reaction, will serve to hinder your efforts. This demo will test your wits, strength, and help you learn along the way.
Nitrogen Discharge Laser
Sasha Zhdanova’s group
Lasers are everywhere – in your CD-ROM drive, in the pointer you use for presentations, in the scope of the rifle you use at the range. This is a demonstration of the “simplest” lasing medium – the nitrogen in the air. Our pump is an electric discharge from a 10 kV power supply, our cavity is the space between two parallel metal plates. The UV light from the laser is powerful enough to ignite a piece of paper, demonstrating both how lacking our eyes are in terms of viewing the electromagnetic spectrum and the processes involved in making many of our modern devices work.
Non-Inertial Frames
D. Heshani Jayatissa’ group
When a container of water is at rest (in an inertial reference frame) the surface of the water is flat (horizontal). However, when the container of water is placed on a rotating platform and spun at a constant angular velocity, the water height forms the shape of a parabola due to the centrifugal force in the rotating (non-inertial) reference frame. This demonstration uses a table tennis ball suspended in water to show how they will behave in such a frame of reference. When this spinning body of water is also accelerated along a straight line, the non-inertial behavior is more prominent.
Oersted’s Effect
John Mason’s group
The link between Electricity and Magnetism Have you ever wondered what links the electricity that powers the light in your house to the magnet you can find on your fridge? In fact, electricity and magnetism are one in the same. We have built an apparatus that demonstrates the connection between electricity and magnetism. Students will notice that when the light bulb is illuminated, the direction of the compasses will change. In fact, the direction in which the compass points is entirely dependent upon its orientation with respect to the current-carrying wires.
Optical Illusions
Mariia Shutova’s group
What will happen when your brain can’t believe your eyes and changes the reality? It is happening all the time! If you look at an optical illusion, you may think you’re seeing things — such as a curved line that’s actually straight, or a moving object that’s standing still. It is your brain that is playing tricks and trying to interpret the image you see. The demonstration shows the most exciting optical illusions that ever existed and explains why we are fooled by our brain.
Paramagnetic Oxygen
Tyler Morrison’s group
This demonstration focuses on the paramagnetic nature of liquid oxygen. Liquid oxygen is poured over a strong set of magnets generating an intense magnetic field. Due to the paramagnetic (“likes to be near magnets”) nature of liquid oxygen it will stick to the magnets until it evaporates.
Phonebook Friction
Dawson Nodurft’s group
The interlocking of pages between two phonebooks allows for a substantial frictional force when attempting to pull the two apart. We challenge people to attempt this by challenging their friends to a tug-of-war!
Photoelectric Effect
Sriteja Upadhyayula’s group
Albert Einstein was awarded the Nobel Prize in Physics for the discovery of the photoelectric effect. In the photoelectric electrons are emitted from either a solid or a liquid when it is hit by photons of energy higher than the electron binding energy in the material. This will be demonstrated using an ultraviolet light source and aluminum foil/cans.
Photoelectric Effect
Sriteja Upadhyayula’s group
Albert Einstein was awarded the Nobel Prize in Physics for the discovery of the photoelectric effect. Photoelectric effect is when electrons are emitted from either a solid or a liquid when it absorbs light. This will be demonstrated using an ultraviolet light source and aluminum foil/cans.
Photoelectric Effect Demonstration
Anton Shutov’s group
Want a great way to compete with your friends while learning some modern day usages for physics principles of light? The photoelectric effect demonstration allows adults and children to compete to see who can fill their bucket to the top and trip the laser that releases all the water. First to trip the laser, wins! A great and fun demonstration of photoelectric sensors.
Piezoceramic Demonstration
Sasha Zhdanova’s group
Piezoceramics are like piezoelectrics except they work much better because they are manufactured, and not found in nature. Some crystals and man-made materials have the property of converting stress in the crystal (i.e. mechanical energy like a thrown object or people stomping) directly to electricity. They are currently used in a variety of places, from cigarette lighters to dance floors. We demonstrate piezoelectric properties by dropping (or throwing) a ball on a piezoceramic and watching it light up a neon lamp if you throw it hard enough – fun for all ages!
Plasma Light Demo
Elizabeth Sooby’s group
The fluorescent light is an everyday example of how we use plasma. The tube is filled with argon and a small amount of mercury vapor. When a voltage is applied to the electrodes in the light fixture, current is drawn across the gas and produces an argon/mercury plasma. Light is emitted in single colors, like blue or red, not all colors like in a rainbow. These are “discrete” colors (atomic line emission) emitted by the excited electrons in the gas.
Plasma Speaker
Alexx Perloff’s group
A plasma speaker is a device which uses a controlled electrical arc (plasma) to play music. Conventional speakers, for all of their simplicity and reliability, have some drawbacks. The sound (pressure waves) quality is limited by the inertia of the speaker cone as well as the fatigue on the material of the cone due to the continual vibration used to make the sound. These limitations are not found in plasma speakers, whose sound comes from the vibration of the air molecules around the plasma arc. The plasma’s temperature is varied by a change in voltage, which is in turn controlled by the music. This causes the air molecules around the arc to either expand or contract, creating pressure waves. The benefits of the plasma speaker come from the relatively limitless supply of ionizable gases to make the plasma and their almost negligible weight. The fidelity of the sound is no longer limited by a speaker cone. We have build a plasma speaker, whose roots can be traced back to physicist William Duddell in 1899.
Polarization and Birefringence
Michael Cone’s group
Polarization is a key property of light waves with many interesting manifestations in nature. Both the sunglasses we wear outside, and the 3D glasses we wear in the movie theater owe their functionality to polarization. We also exploit polarization in photography to reduce the glare coming off of reflective surfaces like water, or glass. On the other hand, Birefringence is a property of some materials that cause different polarizations of light to travel at different speeds inside the material. Calcite crystals, and the double images they produce are are one of the quintessential examples of birefringence in nature. This demonstration explores the properties of polarized light and birefringence by allowing one to observe the effects of different polarization conditions.
Potato-Radio
Mariia Shutova’s group
Everybody knows about radio waves transmitting the music, phone calls and data. But nobody knows that potato can serve as a radio! This is the simplest kind of radio, which can be made at home in several minutes.
Power Through Induction Currents
John Mason’s group
Many of the lights and appliances in your house are powered through an electrical current from the outlet in your wall. While that current is generated from a power plant, electrical currents can be generated very easily at home. By exposing a conductive metal like copper to a changing magnetic field, an electrical current can be generated. If the magnetic field is strong enough, the current generated can even power a light bulb. Our demo will show how a magnet can generate this current by passing a high powered magnet through several copper wire loops and powering several LED light bulbs along the way.
Probing the Nucleus Air Hockey:
Michael Mehlman’s group
In some respects, nuclear and high energy physics is just shooting in the dark! Scientists shoot high energy particles at an unknown and watch what comes out of the collision in order to determine the composition of the target. In the same way, visitors will be instructed to hit an air hockey puck (probe) into a covered area where some unknown shape has been placed (the nucleus). By judging how the puck bounces off the hidden shape, the visitor can make a guess as to what is under the cover, giving them a simple analogy to how many particle physics experiments work!
Renewable Energy Demonstration
Elizabeth Sooby’s group
Solar panels, wind turbines, and hand crank generators, OH MY! There are several ways to power our daily devices. We have a hand crank generator, wind turbine, and a solar panel to demonstrate the conversion to electrical power from the energy generated by each of these renewable sources! We have a cell phone charger, lamp which you can try to power with the hand crank generator. There are volt and amp meters connected to the output of the wind turbine, which is powering an LED light. Finally we have solar panel cars you can power with a laser pointer.
Rodin Coil
Ellie Figueroa’s group
Alternating current is run through a loop, creating a very strong magnetic field inside of the coil. When magnetic objects are placed in the center of the coil, the magnetic field created by the coil causes the magnets to rotate and even levitate.
Second Harmonic Generation
Dawson Nodurft’s group
Have you ever wondered how you get the green light from your laser pointer? Semiconductors when set up properly can create light when stimulated with the application of voltage. The problem is that the light that semiconductors can make doesn’t give off the 532 nm light that we see. So how do we get it? Through second harmonic generation! The addition of a crystal that the infrared light passes through takes the 1064 nm light from the laser and cuts the wavelength in half!
Solar Powered Cars
Michael Mehlman’s group with Elizabeth Sooby’s group
In this demo, visitors will shine a high-powered flashlight at a solar powered car placed on a track. With two or more vehicles, visitors can race the cars around the track… the one who hits their car most directly with the flashlight, thereby imparting the most energy, will win the race! This teaches visitors about how light (an electromagnetic wave) carries energy, and also touches on sustainable energy sources.
Sonoluminescence
Sriteja Upadhyayula’s group
This demo will show how sound/pressure waves cause a bubble in a viscous fluid to collapse on its own and give out bright flashes of light. In the process the bubble interior can get very hot: over 10,000 K.
Another demo we are working on will again use sound to produce light. Here the sound waves will hit a detector, which will power a series of LED lights, producing light. This is an exercise in wireless power transmission.
Sonometer
Sasha Zhdanova’s group
This musical experiment is an instrument that only a physicist could love. It is a simple but versatile demonstration of what causes stringed instruments to make their characteristic sounds. A guitar string is suspended over a hollow wooden box, and pulled taut by free-hanging weights. By increasing the weight on the end of the string, the tension is increased, and the pitch of the sound that the sonometer produces increases. By moving a bridge positioned on top of the string, the string’s effective length changes, which also affects the pitch. These changes can be heard easily, by simply plucking or bowing the string. This interactive demonstration shows the physical factors that go into creating the sound frequencies we hear all the time in music.
Sound Wave Oscilloscope
Dawson Nodurft
Ever want to see the oscillation caused by a sound wave in your ear? Using a can, simple latex membrane with a mirror glued on it, and a laser beam, we can visualize motion of a sound wave like on an oscilloscope! Interesting patterns emerge!
Spacetime Simulator
Jonathan Perry’s group
Every object in the universe, which includes black holes, stars, and planets, tend to warp space time in such a way that it exerts a pull on other objects. As two objects get closer to one another, this pull (and the warping of spacetime) increases greatly. If a smaller body has sufficient speed, instead of colliding with the larger object it will move either an open or a closed path. A closed path also goes by the more familiar name: orbit. Our hands on simulator will showcase a variety of potential space time configurations and demonstrate how objects (such as planets, moons, asteroids, and comets) behave as they circle a much more massive central object.
Spark Detector
Michael Mehlman’s group
In this demo, a high voltage is placed between some wires and a grounded electrode. When an alpha emitter is placed near the wires, sparks start to jump between wires and the electrode. The alphas temporarily ionize some of the air in this region, creating an easier path for electricity to flow!
Still Waters
Ellie Figueroa’s group
By syncing the frequency of vibration of falling water to that of a camera, water gives the illusion of not moving at all, and, in some cases, going upwards.
Stroboscopic Effect Fan Demonstration
Anton Shutov’s group
How fast can your eyes see movement? With a simple strobe light and a cleverly decorated fan, we are able to see the effects that sudden bursts of light, at the right emission frequencies, can trick our brains into seeing stopped fan when it is actually not. Best of all, it’s an experiment you can make at home and show your friends!
Supersonic Ping-Pong Ball Cannon
Dawson Nodurft’s group
We show how to accelerate a ping pong ball to supersonic speeds by simply evacuating a tube of its air. By catastrophically destroying one of the aluminum foil ends to allow the air back in the ping pong ball is accelerated by almost 5,000 g’s. We attain supersonic velocity by putting compressed air at one of the ends of the cannon. The addition of this causes extra force to be placed on the ping pong ball giving it the speed needed to go through an aluminum can or dent a wood block.
Temperature Effect of Resistance
Tyler Morrison’s group
Resistance of most materials decreases with decreasing temperature. To demonstrate this effect a light bulb is connected in series with a coil of very fine copper wire and a DC power supply. The voltage is adjusted so that the bulb glows dimly. When the coil is immersed in liquid nitrogen the very fine copper wire will cool and the resistance of the wire decreases causing the current to increase and the bulb to glow brightly.
Tesla Coil
Andrea Delgado’s group
The Tesla Coil has played a significant role in stimulating generations of students to become interested in the wonders of science and electrical phenomena. Developed around 1891 by the inventor Nikola Tesla, the TC can be used to produce high-voltage, low-current, high-frequency, alternating-current electricity. Tesla used the TCs to conduct experiments involving the transmission of electrical energy without wires to extend the limits of our understanding of electrical phenomena. Generally speaking, an electrical transformer transfers electrical energy from one circuit to another through the transformer’s coils. A varying current in the primary coil winding creates a varying magnetic flux in the transformer’s core, which then creates a varying magnetic field through the secondary winding that induces a voltage in the secondary winding. In the TC, a high-voltage capacitor and spark gap are used to periodically excite a primary coil with bursts of current. The secondary coil is excited through resonant inductive coupling. The more turns the secondary winding has in relation to the primary winding, the larger the increase in voltage. Million of volts can be produced in this fashion. Although not all commercial applications for Tesla coils are still in use, some historical and modern day applications include: spark gap radio transmitters, induction and dielectric heating, induction coils, medical X-ray devices, ozone generators, particle accelerators, and basically any device that relies on generation of extremely high voltages with relatively high power levels.
The Lever Demonstration
Ellie Figueroa’s group
As you pull down on the rope a force is applied downward that lifts the sandbag on the other end of the lever. This downward force is called Torque. As you apply torque at a further distance from the fulcrum, it becomes easier to lift the bag of sand.
The Power of Circuit Design
John Mason’s group
Everything: your cell phone, television, even your computer, are highly dependent upon an ability to design a complex circuit. Each of these devices is run distinctively by controlling the current and voltage generated by an electrical source. We are working to generate a demonstration, which brings to life the magic of circuit design of a complicated LED system.
Students will be able to choose the path of the current and voltage by choosing from a number of switches to illuminate a number of constellations. Through this demo, the power of circuit design is demonstrated while viewing some of the world’s most famous and recognizable constellations.
Ultra-Sonic Acoustic Levitation
Michael Cone’s group
In this demonstration we show how one can levitate small objects in midair using the pressure from focused sound waves. We use an ultra-sonic transducer combined with a slightly concave reflecting surface to create a standing wave at around 27,000 Hz. These ultra-sonic sound waves are outside the range of human hearing, but we can “see” their presence by levitating small low-density objects. Specifically, the objects (styrofoam beads in this case) sit slightly below the nodes of the pressure waves. For these points the downward force of gravity is balanced by the upward force provided by the pressure waves. We can also use the vapor from liquid nitrogen to visualize the nodes and anti-nodes of the standing waves.
Volta’s Hailstorm and Franklin’s Bells
Landon Chamber’s group
The electric potential difference produced by a Wimshurst generator can produce energy in the form of motion and sound. When two metal plates, each at a different potential, close-off a chamber containing pith balls, a potential difference is set up within the chamber. The charges in the pith balls will separate, causing them to be attracted to one of the plates. When the pith ball strikes a plate, the overall charge of the pith ball changes. The pith ball is repelled away from the plate and attracted to the opposite plate. The process continues for many pith balls, creating a “hailstorm” of pith balls tossing around the chamber. In another demonstration, the potential difference can be applied across two adjacent bells with a metal ball hanging between them. By the same physical effect, the metal ball will ring both bells repeatedly. This noisy demonstration served as an alarm for incoming electrical storms in 19th century households.
Vortex Coils
Ellie Figueroa’s group
We create strong magnetic fields inside a wire vortex such that, by placing a magnetic ball in the middle of it, the ball starts spinning even though nothing seems to be touching it.
Walk Through The Solar System
Leo Alcorn and Peter Chi’s group
Students at Texas A&M have been working to design and build a permanent scaled walkway of the Solar System. Interesting information about the Sun and each of the 8 planets are depicted on mounted plaques containing a visual and written description. The walkway is scaled to be about 280 billion times smaller than our solar system. If you shrunk a school bus by that much, it would be smaller than the size of an atom! This walkway will aid students and the community in understanding our solar neighborhood and the vast distances between objects in the cosmos.
Why Don’t Planets Twinkle?
Leo Alcorn and Peter Chi’s group
Stars twinkle in the night sky due to the scattering of their light by turbulent motion of air in our atmosphere. But why doesn’t the light reflected from planets or the moon appear to twinkle? This demo answers that question while also displaying multiple other fundamentals of observational astronomy. We use lasers to show how light coming from a single point, similar to how we see light from distant stars, will jitter (or twinkle) when exposed to turbulent air. However, lasers with a wider beam width, resembling the more extended light from planets or the Moon, do not produce a jittery image since the fluctuations become averaged over the wider image. Then, we use these lasers to demonstrate the path light takes through a telescope to produce an upside-down image. Finally, we also show how astronomers use filters to block specific colors of light, to study the full spectrum of the Universe.
Wireless Energy Transfer
Elizabeth Sooby’s group
Who needs power cords? Via electromagnetic induction, power can be transferred from one circuit to the next. This demonstration powers an LED light wirelessly. The primary circuit is driven by a function generator. Similar technology enables wireless charging of phones, cars, and much more!